Institute of Oceanology, Chinese Academy of Sciences
Article Information
- NIU Jiaohong, SUN Chengjun, YANG Bo, XIE Lei, JIANG Fenghua, CAO Wei, CHEN Yan, DING Haibing, HUANG Yuhuan, GAO Xianchi
- Vertical variations and composition of dissolved free amino acid in the seawater of the Yap Trench in the western Pacific Ocean
- Journal of Oceanology and Limnology, 41(1): 118-137
- http://dx.doi.org/10.1007/s00343-022-0190-x
Article History
- Received May 10, 2020
- accepted in principle Jun. 28, 2020
- accepted for publication Jan. 6, 2022
2 Marine Ecology and Environmental Science Laboratory, Pilot National Laboratory for Marine Science and Technology(Qingdao), Qingdao 266237, China;
3 Marine Ecology Center, the First Institute of Oceanography, Ministry of Natural Resources, Qingdao 266061, China;
4 Qingdao No. 2 Middle School, Qingdao 266100, China;
5 College of Chemistry and Chemical Engineering, Ocean University of China, Qingdao 266100, China;
6 Qingdao Collaborative Innovation Center of Marine Science and Technology, Ocean University of China, Qingdao 266100, China
Amino acids (AAs) are important reservoirs of organic carbon and organic nitrogen in the ocean, and are also highly susceptible to marine organisms. Concentrations of amino acids are significantly affected by marine biological and biochemical processes, such as releasing and uptaking of plankton and microorganisms, photosynthesis, respiration, etc. (Cowie and Hedges, 1994). In seawater, amino acids can be separated into dissolved amino acid (THAA) and particulate amino acid (PAA). THAA accounted for about 10% of dissolved organic nitrogen (DON) and PAA accounted for 49%±20% of total organic nitrogen (TON) (Dittmar et al., 2001). There are two forms of TFAA in seawater—dissolved free amino acid (DFAA) and dissolved combined amino acid (DCAA). DFAA is not only an important nitrogen source for phytoplankton (Burdige, 1991), but also an important nitrogen and carbon source for heterotrophic organisms such as bacteria (Veuger et al., 2004). The variation of DFAA concentrations is affected by many factors, including biomass and biological metabolism, seawater movement, sediment resuspension, organic matter degradation, etc. (Hamilton, 1984). DFAA is also an important intermediate product of marine nitrogen cycle, which can be directly converted to organic acids and ammonia-nitrogen by deamination of microorganisms (Behrends and Liebezeit, 1999). Studying the variations, distributions and controlling factors of concentrations of DFAA in seawater will contribute to understanding the organic nitrogen and carbon cycles in the ocean. Until now, a series of studies have focused on the characteristics of DFAA in surface seawater (Table 1). However, the distribution of DFAA in the abyss and hadal zone is yet to be explored.
![]() |
In general, the ocean abyss and the hadal zone are between 4 000 m and 6 000 m and below 6 000 m from the sea surface, respectively. The Yap Trench is one of the deepest trenches in the world. It is located in the Western Pacific Ocean Yap Ridge and the eastern side of the Yap Islands. The trench is in a complex geological structure area among the Philippine Plate, the Caroline Plate and the Pacific Plate, forming a "J" shape. Its deepest point is 8 527 m. In the last several decades, a series of studies had been conducted on its physical, biological, and geological characteristics (Hawkins and Batiza, 1977; Kaneko et al., 1998; Fujiwara et al., 2000). Recently, several Chinese projects have targeted the Yap Trench and several valuable findings have been reported (Yan et al., 2020). The composition of sediments and morphology of rocks in the trench (Yue et al., 2018; Huang et al., 2020), the seabed heat flow (Song et al., 2016) etc., in the trench were investigated. However, few studies focused on the biogeochemical characteristics of the seawater AA in the trench. In this study, the DFAA in the seawater from the northern region of the trench was qualitatively and quantitatively analyzed. Vertical variations of the concentrations of DFAA and the amino acids from the euphotic to hadal zone of the trench were explored and the factors controlling the compositions and concentrations of the DFAA were discussed. This is the first study regarding DFAA from sea surface to hadal zone in the Yap Trench. These results help to understand the organic nitrogen and carbon cycles from sea surface to the hadal zone.
2 MATERIAL AND METHOD 2.1 Sampling areaFrom May 13 to May 23, 2016, R /V Xiangyanghong 09, carrying "Jiaolong" manned submersible, conducted the No. 37 ocean cruise in the northern region of the Yap Trench. The shipborne Seabird CTD equipment was used to collect seawater from four CTD stations in the study area. Five dive stations located on the sediment-seawater interface, were set inside of the trench. Stations Dive 109, Dive 110, Dive 111, and Dive 112 were on the western side of the trench, and Station Dive 113 was on its eastern side. The overlying water from the sediment-seawater interface of the dive stations was collected by SBE-911 plus CTD (Seabird Electronics) which was carried and controlled by "Jiaolong" manned submersible. Parameters such as seawater temperature, salinity, and water depth were measured simultaneously by CTD when collecting seawater samples. The sampling site map is shown in Fig. 1, and the information of the stations is shown in Table 2.
![]() |
Fig.1 Location of sampling stations in the Yap Trench PHS: the Philippine Sea plate; PAC: the Pacific plate; CP: the Caroline plate; MT: the Mariana Trench; YT: the Yap Trench; PT: the Palau plate; CR: the Caroline Ridge. |
According to the modified method by Lindroth and Mopper (1979), the o-phthalaldehyde (OPA) and 3-mercaptopropionic acid (MPA) precolumn derivatization high-performance liquid chromatography (HPLC) was applied to determine concentrations of DFAA in seawater. The operational parameters were: injection sample volume was 20 μL, the flow rate was 0.4 mL/min, column temperature was 33 ℃, fluorescence detection excitation wavelength (λex)=337 nm, and emission wavelength (λem)=454 nm. The instrument was Waters e2695 HPLC system with a quaternary gradient pump, online degasser, Agilent ZORBAX Eclipse Amino Acid Analysis Column (150 mm×3.0 mm, 3.5 μm), and e2475 fluorescence detector.
The mobile phase A was 0.05-mol/L sodium acetate buffer solution (pH=7.2±0.05; sodium acetate solution꞉ tetrahydrofuran (V/V)=100꞉1); and the mobile phase B was acetonitrile꞉methanol꞉Milli-Q (Millipore Elix10 USA) (V/V/V)=1꞉1꞉1 mixture. The two mobile phases were filtered through a 0.2-μm PALL Supor-450 filter.
240 μL of derivatization reagent (OPA-MPA) was added to 760 μL of seawater sample (filtered with 0.7-μm Whatman (GF/F) glass fiber filter). After 19 min of derivatization, 20 μL of the derived sample was injected into the HPLC. The amino acids were separated based on the elution gradient (Table 3). The concentrations of various amino acids were determined by comparing peak areas of HPLC profiles between the sample and the amino acid standards. Fourteen amino acids were identified in the seawater samples from the sampling stations, including two acidic amino acids (Asp: aspartic acid, Glu: glutamic acid), ten neutral amino acids (Ser: serine, Gly: glycine, Thr: threonine, Ala: alanine, Tyr: tyrosine, Val: valine, Met: methionine, Phe: phenylalanine, Ile: isoleucine, Leu: leucine) and two basic amino acids (Arg: arginine, His: histidine). Concentrations of the DFAA were obtained from the sum of all amino acids. All chemicals and reagents were of the highest available grade purchased from Sigma.
A method blank (for DFAA determination) was obtained by injecting Milli-Q water (equal volume with sample) with the derivative reagent using the same sample protocol. The reagent blank was determined once a day. Our result showed that DFAA was not detected in the method blank. The average deviation of all amino acids was ±0.5% (at 80 pmol levels), and the detection limit was 60 fmol.
2.2.2 Determination of environmental parameters of the seawater samplesThe parameters about temperature (T), pH, salinity (S), concentration of dissolved oxygen (DO), dissolved inorganic nitrogen (DIN), and chlorophyll a (Chl a) were obtained from the unpublished data of the Second Institute of Oceanography and the National Deep Sea Center, Ministry of Natural Resources.
T, S, and concentration of DO were measured synchronously in seawater sampling; pH was determined within 24 h by spectrophotometric method (Dickson et al., 2007). About 250-mL seawater (filtered with 0.45-μm cellulose acetate filter) was stored at -20 ℃ for analysis the concentrations of DIN after returning to the shore-based laboratory. Concentrations of nutrients were determined by Seal Analytical AA3 automatic nutrient analyzer (Strickland and Parsons, 1972).
The concentration of Chl a in the seawater samples was analyzed by fluorescence spectrophotometric method (Hamilton, 1984). The seawater samples (300 mL) were filtered through the Whatman GF/F glass fiber filter, and then the filter was wrapped in aluminum foil and stored at -20 ℃. During the analysis, the filter was immersed in a dark place with 90% (volume fraction) aqueous acetone solution for 24 h. The solution was measured by using a fluorometer (F-4500, Hitachi Ltd., Japan).
2.2.3 Calculation of degradation index (DI) of DFAAThe amino acid spectra of the 70 samples were used in a variance-oriented method (Principal Component Analysis, PCA) to derive the principal components, which obtained several new principal components through dimension reduction transformation. The first principal component was used for DI calculation to indicate the degradation degree of organic matter. Based on the formulas provided by Dauwe and Middelburg (1998), the DI values were calculated by principal component analysis:
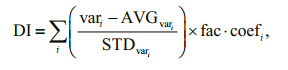
where vari is the original (non-standardized) molar percentage of amino acid i; AVGvari and STDvari are its mean and standard deviation; fac.coefi is coefficient factor. The coefficient factor was obtained by SPSS (Statistical Product and Service Solutions) software. If the DI was positive, the higher of the value, the organic matter was fresher, indicating that DFAA mainly came from the newly produced organic matter of phytoplankton. In contrast, if the DI was negative, its higher absolute value showed the organic matter in the seawater was older, and may indicate a greater extent of DFAA degradation.
3 RESULT 3.1 Vertical variations of physical and chemical parameters in seawater of the northern region of the Yap TrenchHydrology, physical, and chemical factors not only affect the natural distribution of biogenic elements, but also play an important role in the selective utilization of organic matter by organisms, and affect its metabolic pathways. The vertical profiles of these factors are shown in Fig. 2. The temperature decreased with water depth, and the thermocline appeared between 100 m and 150 m. The oxygen minimum zone (OMZ) occurred in the mesopelagic layer (200 m and 1 000 m), with minimum values of 58.65– 94.50 μmol/L between 500-m and 1 000-m depth. Below 1 000 m, the concentration of DO gradually increased with water depth. The concentration of DIN increased from sea surface to 1 000 m, and then slightly decreased with depth. For the seawater samples from the four stations, the values of pH ranged from 7.8 to 8.3, with the lowest values around 1 000-m depth. The vertical variation profiles of Chl a were relatively stable in the euphotic layer at the CTD04 station. For the other three stations (CTD0–CTD03), above 100 m, the concentrations of Chl a increased with depth; and then decreased with depth below 100 m. In general, T, S, pH, and concentration of DIN were relatively stable from the bathyal layer to the hadal zone.
![]() |
Fig.2 Vertical profiles of T, pH, S, concentrations of DO, Chl a, and DIN in the CTD01–CTD04 stations The data of T, pH, S, DO, DIN, and Chl a were from the unpublished data of the Second Institute of Oceanography and the National Deep Sea Center, Ministry of Natural Resources. |
Overall, the concentrations of DFAA in the seawater of the study area decreased with depth, ranging from 0.05 to 1.78 μmol/L. The maximum concentration of DFAA appeared at 10-m depth (1.78 μmol/L) of the CTD04 station, and the minimum concentration occurred at 125-m depth (0.05 μmol/L) of the CTD03 station (Table 4). The mean values of concentrations of DFAA in different water layers of the trench are listed in Table 5. In the study area, the average concentration of DFAA reached the maximum in the mesopelagic layer (200–1 000 m), and reached the minimum in the bathyal layer. From the bathyal layer to the abyssal layer of the trench, the concentrations of DFAA increased gradually.
![]() |
![]() |
The vertical variation trends of concentrations of DFAA in the seawater of the four CTD stations were roughly similar (Fig. 3). In the study area, from the euphotic layer to the mesopelagic layer, the variations of concentrations of DFAA were complex. From the bathyal layer to the abyssal layer, the concentrations of DFAA were relatively stable. For example, in the euphotic layer of station CTD01, the concentrations of DFAA increased from surface seawater to 75-m depth and then decreased to 200-m depth. In the mesopelagic layer, the concentrations kept increasing to 800-m water depth and dropped obviously to 1 000-m depth. At station CTD02, the concentrations of DFAA in the seawater fluctuated slightly with depth, ranging from 0.13 to 0.48 μmol/L. The concentrations of DFAA had two concentration thermoclines in the seawater of station CTD03. One was located around 500-m depth, and the concentrations increased significantly, up to 1.16 μmol/L. The other one appeared at 50-m depth. Below that depth, the concentrations of DFAA decreased remarkably to the minimum (0.05 μmol/L) at 125-m depth. The vertical variation profiles of the concentrations of DFAA in the seawater of station CTD04 only had one thermocline around 10-m depth. At that depth, the concentrations reached the maximum (1.78 μmol/L).
![]() |
Fig.3 The vertical profiles of concentrations of DFAA in the seawater from CTD01 to CTD04 stations |
The concentrations of DFAA in the seawater samples from sediment-seawater interface (stations Dive 109, Dive 110, Dive 111, Dive 112, and Dive 113) are shown in Fig. 4. Stations Dive 109–Dive 112 are located on the western side of the trench. The concentrations of DFAA in the seawater samples from Dive 110 to Dive 112 stations (which are close to or in the hadal zone) were higher than those in the bottom layer of the four CTD stations. The highest concentration was found in station Dive 111 (0.80 μmol/L), and the lowest concentration was only 0.20 μmol/L in station Dive 113, located on the eastern side of the trench. In the study area, the concentrations of DFAA of the seawater sample from the seawater-sediment interface were higher than those from the water column at similar water depth. For example, the concentration of DFAA was 0.13 μmol/L at the depth of 6 000 m in station CTD02, while in the adjacent station Dive 110, the value was 0.56 μmol/L. The concentration of DFAA was 0.43 μmol/L at the depth of 5 672 m in station CTD03, while in the adjacent station Dive 112, the value was 0.59 μmol/L.
![]() |
Fig.4 The average concentrations of DFAA in different layer of the four CTD stations and the concentrations of DFAA in the five dive stations |
Overall, the vertical variation trends of concentrations of the 14 amino acids in the seawater of stations CTD01–CTD04 were similar as those of DFAA (Supplementary Fig.S1). In general, their higher concentration occurred in the euphotic layer, and then decreased with depth. The concentrations of the amino acids varied remarkably in the euphotic and mesopelagic layers, and varied slightly in the bathypelagic and abyssal layers of the trench. In the seawater samples of the four CTD stations, most of the highest values of the concentrations appeared in the euphotic and mesopelagic layers in the four stations (Table 6).
![]() |
The molar percentages of the 14 amino acids in different water layers of the northern region of the Yap Trench are shown in Fig. 5a–e. At different stations, the dominant amino acids were different in each layer. For example, at station CTD01, Asp and Glu were dominant in the euphotic and mesopelagic layers, and Gly was dominant in the abyssal layer. However, the molar percentage of Glu was extremely low in the bathypelagic and abyssal layers of all the four stations, and close to 0 in stations CTD01 and CTD02. Gly had the highest molar percentage in the bathypelagic layer of station CTD02, in the abyssal layer of station CTD03 and in the mesopelagic layer of station CTD04. In the abyssal layer, the molar percentage of His was the highest (23.80%) at station CTD02 but low at CTD03 (5.72%). As a comparison, the molar percentage of Ser was low at station CTD02, but it was up to 21.80% at station CTD03. Ile only existed in the euphotic and mesopelagic layers of station CTD03, with a molar percentage less than 0.50%. At the seawater-sediment interface, the molar percentage of Val was the highest (32.40%) in the seawater of station Dive 109 (Fig. 5e). At stations Dive 110, Dive 111, and Dive 112, Ser and Thr were the dominant amino acids in the seawater. Their molar percentages ranged from 15.65% to 22.82%, and from 14.49% to 23.67%, respectively. The dominant amino acids were Glu and Val in the seawater from station Dive 113, with molar percentages of 20.54% and 19.96%, respectively. At the five stations, Ile was not detected in all the seawater samples.
![]() |
Fig.5 The molar percentages of various amino acids of DFAA in the euphotic, mesopelagic, bathypelagic, and abyssal layers in stations CTD01–CTD04 (a–d) and stations Dive 109–Dive 113 (e) |
In the seawater of the study area, neutral amino acids occupied the largest fraction of DFAA, and basic amino acids occupied the smallest fraction. The concentrations of acidic, neutral, and basic amino acids of DFAA varied significantly in the seawater samples. At station CTD01, the high concentrations of acidic amino acids appeared at 75 m and 800 m (Supplementary Fig.S2a). The concentrations fluctuated slightly with water depth at stations CTD02, CTD03, and CTD04. In the euphotic layer, the concentrations of basic amino acids at station CTD01 tended to be stable with depth. In contrast, the concentrations showed strong fluctuations at stations CTD02, CTD03, and CTD04 (Supplementary Fig. S2b). For neutral amino acids, the variation profiles of their concentrations in each station showed complex patterns. At station CTD01, a concentration thermocline appeared at 100-m depth, with the highest value 0.60 μmol/L. At station CTD04, the dramatic increase of the concentrations of neutral amino acids occurred from sea surface to 10-m depth, increasing from 0.39 to 1.50 μmol/L (Supplementary Fig.S2c).
The molar percentage of acidic amino acids decreased in the order of stations Dive 109, Dive 113, Dive 110, Dive 112, and Dive 111, and the trend was almost reversed for the neutral amino acids (Fig. 6). The highest molar percentage of acidic amino acids appeared at station Dive 109 (30.12%), and the lowest value was 14.82% in station Dive 111. Neutral amino acids occupied the largest fraction of DFAA in all five stations. At station Dive 111, the molar percentage of neutral amino acids was as high as 80.21%. At station Dive 109, the value was the lowest but still exceeded 50%. The basic amino acids had the lowest molar percentage, with a maximum value at station Dive 109 (14.84%), and a minimum value at station Dive 111 (only 4.97%).
![]() |
Fig.6 The molar percentages of acidic, basic, and neutral amino acids of DFAA at the five dive stations |
In the four CTD stations, the DI of DFAA in the seawater varied remarkably in the euphotic layer and the mesopelagic layer, ranging between -3.13 to 1.46, with maximum value at 500-m depth in station CTD03 and minimum value at 500 m in station CTD01, indicating that the sources and degradation behaviors of organic matter in the euphotic and mesopelagic layers were significantly different (Fig. 7). Below 1 000-m depth, the DI values became relatively stable. In the five dive stations, the DI values ranged from -0.86–0.56 (Table 7), with the lowest value at station Dive 109 and the highest value at station Dive 112.
![]() |
Fig.7 Vertical variation profiles of DI in CTD01–CTD04 stations |
In the marine environment, the concentrations of DFAA varied significantly in different areas (Table 1). Previous studies showed that the concentrations of DFAA in seawater ranged from 0.002 to 1.960 μmol/L (Clark et al., 1972; Lee and Cronin, 1982). In general, the concentrations of DFAA were relatively higher in coastal waters, but our results were in a comparable range to other open ocean areas: 0.05 to 1.78 μmol/L (the concentration of DFAA less than 0.64 μmol/L accounted for 86% of seawater samples) in this study, compared to 0.36 to 0.64 μmol/L in the Arctic waters (Hubberten et al., 1995) and 0.28 to 0.60 μmol/L in the Antarctic waters (Hubberten et al., 1995). The high concentration of DFAA occurred in the euphotic layer, the mesopelagic bottom layer and the abyssal layer (Fig. 3). The results are consistent with the high concentration of dissolved organic carbon (DOC) (unpublished) in the Yap Trench.
Above the mesopelagic layer (< 1 000 m), the variations of concentrations of DFAA are complex. In the study area, the average concentration of DFAA in the euphotic layer (0.48±0.37 μmol/L) is slightly higher than that in the northeast Atlantic Ocean (0.31 μmol/L) (Lee and Cronin, 1982). Keeling and Revelle (1985) reported that a barrier layer formed between the base of the shallow halocline and the top of the thermocline suppressed the upward flux of nutrients in the Western Pacific Warm Pool (WPWP), which resulted in oligotrophic in the euphotic layer. However, the previous study showed a Chl-a concentration maximum formed in the barrier layer (25–125 m) at the intersection of sufficient light and nutrients (Matsumoto et al., 2004). Their result is consistent with this study that the high concentration of Chl a occurred at 100 m in the euphotic layer. On the other side, there was no significant correlation between DFAA and Chl a in this study, indicating that photosynthesis is not the only factor controlling concentrations of DFAA in the seawater of the euphotic layer in the study area. Previous studies also showed that the relationships between Chl a and DFAA in different sea areas were complicated (Chen and Yang, 2010). In fact, DFAA concentrations are closely related to the photosynthesis of phytoplankton and assimilation of heterogeneous bacteria in the euphotic layer (Burdige, 1991). Jørgensen et al. (1993) found that dissolved DFAA and NH+4 may be the major sources of N to aquatic bacteria. In this study, the variation trends of vertical profiles of DFAA are consistent with that of NH+4 (Fig. 8), but there was also no significant correlation between them, further indicating that multiple factors affect the concentration of DFAA in the euphotic layer. Several studies have shown that the input of terrigenous organic matter exists in different trenches in the Pacific Ocean, such as the New Britain Trench, the Palau Trench, and the Mariana Trench (Luo et al., 2018, 2019; Xiao et al., 2020). Yue et al. (2018) detected that there was terrigenous input of organic matter in the sediments of the Yap Trench, and Guo et al. (2018) found that organic matter in the euphotic layer could be transported to the abyssal seawater through food chain or sedimentation. These findings provide indirect evidence about the existence of terrigenous organic matter in the euphotic layer of the Yap Trench. Kobayashi (2000) reported the distance of the Yap Island Arc, the Yap Trench axis is abnormally short. Station CTD01 is closer to the Yap Island Arc, so it is more likely to be affected by the Yap Island Arc. The results of amino acids from the Atlantic Ocean (Wedyan and Preston, 2008) and the Pacific Ocean (Matsumoto and Uematsu, 2005) showed that some of them enter the open ocean through atmospheric deposition. The wind blew amino acids from the land soil or even island soil over the ocean, and then they sank into the surface seawater by the atmosphere deposition (Scheller, 2001). Mopper and Zika (1987) reported that high concentrations of DFAA (6.50 μmol/L) existed in seawater after precipitation, providing another hint of atmospheric deposition of amino acids to the seawater. In general, the variations of DFAA in seawater of the euphotic layer in the study area are a combination of various processes, including photosynthesis, respiration, and terrigenous input.
![]() |
Fig.8 Variation trends of NH4+ and DFAA at stations CTD01–CTD04 in the seawater of the euphotic layer |
The photosynthesis does not occur below 200 m in the seawater of the Yap Trench. In this study, the concentration of DFAA in the mesopelagic layer was slightly higher than that in the euphotic layer. The concentration of DFAA in the seawater of the mesopelagic layer was mainly related to the degradation of dead organisms, the breakdown of living cells, the sinking of particle organic matters, the assimilation of exotic bacteria, etc. (Behrends and Liebezeit, 1999). Previous studies have shown that amino acids were firstly and mainly from the hydrolysis by aminopeptidases in the low oxygen water of the Gulf of Mexico and the North Atlantic Ocean, and a part of them formed DFAA in the mesopelagic layer (Liu and Liu, 2018). The contribution of free peptidases to total hydrolysis was more than double in organic-rich seawater than that in oligotrophic seawater (Somville and Billen, 1983). Mopper and Lindroth (1982) indicated that the DFAA enrichment occurred in the oxygen minimum zone. In our study, the most significant variations of concentrations and compositions of DFAA were also found at the oxygen minimum zone (800 m) of the mesopelagic layer. This kind of variation might be related to a dramatic change of the environmental factors from the euphotic layer to the mesopelagic layer, leading to the reduction of microbial density and further reduced the utilization of DFAA (Mopper and Lindroth, 1982). Sohrin et al. (2010) found that the biomass of the seawater decreased significantly with the depth in the seawater from the central equator to the subtropical zone of the Pacific Ocean. Because the biomass of water in the mesopelagic layer was much lower than that in the euphotic layer, the biomass consumption and degradation rate of DFAA in this layer should be much less than that in the euphotic layer. Meanwhile, low temperature also decreased the microbial activity and then reduced direct utilization of DFAA in the layer. Wishner et al. (1995) reported the diagenetic transformation, mineralization processes, and degradation by microorganisms in the oxygen minimum zone. However, there was no significant correlation between the concentrations of DFAA and DO, indicating that the degradation of organic matter by microorganisms in the seawater of the mesopelagic layer of the Yap Trench was not the only factor affecting concentrations of DFAA in the layer. Overall, relatively strong degradation of organic matter and weak consumption of amino acids by microorganisms in the mesopelagic layer might cause an increasing concentration of DFAA in the layer of the research area.
In the bathypelagic layer of the study area, the concentrations of DFAA were lower than those in the mesopelagic layer, which might be attributed to decreased input of particulate organic matter. Guo et al. (2018) reported that the particulate organic matter in the seawater of the Yap Trench had undergone bacterial degradation and mineralization processes during the downward transport to deeper water. Meanwhile, the funnel-shaped Yap Trench could promote the migration of particulate organic matter into the abyssal layer, implying a decrease of PAA, which was an important source of DFAA in the seawater (Kirchman and Hodson, 1984). Then, the concentration of DFAA had a declining trend in the bathypelagic layer. In addition, high-latitude cold water masses are an important source of deep-sea seawater (Kaneko et al., 1998). The salinity tends to increase with the depth, and the concentration of DFAA has decreased trend with the depth of the Yap Trench. Similar to DO, there was no obvious correlation between the concentration of DFAA and salinity (R=0.02, P=0.96), indicating that conservative mixing/dilution is not the dominant factor to control the concentrations of DFAA in the bathypelagic layer of the Yap Trench.
The Yap Trench is located in the bathypelagic circulation channel of the Western Pacific Ocean. In the abyssal layer, the concentrations of DFAA ranged from 0.13 to 0.66 μmol/L. Generally, the seawater in the abyssal layer of the Yap Trench originated from the Antarctic Submerged Water (low temperature and high oxygen) which was transported by the circulation of the ocean (Kaneko et al., 1998). Carney (2005) found that the source of DFAA in the abyssal layer was the same as that of the bathypelagic layer, and the utilization of DFAA by microorganisms could be inhibited at a lower temperature in the seawater of the abyssal layer (< 2 ℃). In this study, the variations of concentration of DFAA in the seawater of abyssal layer and sediment-seawater interface showed that the sediment may be the source of DFAA in the seawater of the abyssal and hadal zone of the trench, and the water depth has little effect on them.
4.1.1.3 Variation of concentrations of DFAA in the seawater-sediment interface of the Yap TrenchIn general, in the seawater-sediment interface of the northern region of the trench, the concentrations of DFAA were higher than those in the seawater from the abyssal layer and hadal zone, which might be attributed to the vertical exchange of water body, sediment resuspension, etc. Romankevich (1984) reported that the concentration of dissolved amino acids in interstitial water was higher than that in seawater. The water exchange in the interstitial water and overlying water might lead to the increase of concentrations of dissolved amino acids in the seawater-sediment interface (Wu et al., 2018). In addition, the south-facing bottom water under the action of Coriolis forces scoured the western trench wall more seriously, resulting in resuspension of the sediment, and then part of the PAA being converted into DCAA in the seawater. Some of the DCAA was further hydrolyzed into DFAA (Svensson et al., 2004; Yue et al., 2018). This process might lead to the concentrations of DFAA on the western side of the trench wall being higher than that on the eastern side at a similar depth, providing an explanation for our results that concentrations of DFAA were higher in station Dive 111 and Dive 112 than that in station Dive 113. Overall, the concentrations of DFAA in the seawater from the five dive stations had a similar variation trend with the concentrations of DIN, and had an opposite trend with the concentrations of DO and pH (Fig. 9). With the consumption of DO and organic matter, more CO2 was also produced, leading to a decreased trend of pH values in the seawater. In a word, the different trends of concentrations of DFAA, DIN, and pH in the five dive stations were related to the combining effect of sediment resuspension, organic matter degradation, and the utilization of DFAA.
![]() |
Fig.9 Concentration variations of DFAA, DO, DIN and pH in stations Dive 109–Dive 113 |
In general, the dominant amino acids of DFAA were similar at all the stations in the study area, including His, Gly, Thr, Ala, Glu, and Asp. This similarity occurred in almost all the water layers. Similar results were also detected from the survey of the North Adriatic Sea by Pettine et al. (1999). Studies on the compositions of algae in the ocean showed that these amino acids were the major amino acids constituting algal proteins (Hayashi et al., 1986). Typical amino acids such as Glu could account for 10%–20% of the amino acid content of phytoplankton protein; while Gly was abundant in diatoms, accounting for up to 22.5% of total amino acids. With the death of the algae, these amino acids were adsorbed on the inert cell wall, thereby avoiding their rapid consumption by degradation process. The enrichment of Gly and Thr was not only affected by the release of amino acids after algal cell death, but also affected by their own chemical properties. Compared with other kinds of amino acids, these two amino acids were stable in nature and were not easily degraded by microorganisms or utilized by zooplankton (Middelboe et al., 1995; Preston et al., 1996), leading to their higher concentrations in the seawater samples. However, there are many factors controlling the compositions and concentrations of DFAA in the seawater, and more extensive and deep research is necessary in the future.
4.1.3 Factors affecting the distributions of acidic, neutral, and basic amino acidsIn DFAA, neutral amino acids were the major components in different water depths of the northern region of Yap Trench, of which Ser, Gly, and Thr were dominant. In marine environment, the absorption and utilization of various neutral amino acids by marine microalgae are different (Xu et al., 2013). The concentrations of acidic amino acids were the highest in CTD01 at 75-m depth and CTD01 at 800-m depth. The results were consistent with the vertical variation trends of the concentrations of DFAA. Previous study showed that Glu had a higher concentration in phytoplankton (Braven et al., 1995). Abundant Glu in phytoplankton might be contributed to high concentration of acidic amino acid in both euphotic layer and oxygen-minimum layer. Basic amino acids were considered to be relatively easily absorbed on mineral surface, and have limited degradation by microorganisms in seawater (Li, 2007). In all the four CTD stations, concentrations of basic amino acids increased in mesopelagic layers. It is possible that after utilization of neutral and acidic amino acids in the euphotic and mesopelagic seawater, the absorbed basic amino acids are released into the seawater and then their concentrations increase (Sun, 2012). The enrichment of neutral amino acids in the seawater samples might be caused by the low biomass of phytoplankton in the euphotic layer. After the death of algae, several relatively stable neutral amino acids such as Ser, Gly, and Thr adsorbed on the cell wall might be released into seawater after cell lysis (Zhang et al., 2015).
4.2 Degradation of dissolved organic matter in the seawater of the northern region of the Yap Trench 4.2.1 Tracking organic matter degradation by DI, the molar percentage of amino acids and Tyr/PheAmino acids abundance and component information can effectively reflect the sources of unstable organic matter and its biogeochemical cycle (Davis et al., 2009). The DI of amino acids was widely used to indicate the degradation degree of organic matter (Dauwe et al., 1999; Yamashita and Tanoue, 2003). For example, the DI has been used in assessing the degradation of particulate organic matter in seawater and organic matter in sediments (Dauwe et al., 1999). Since, the fraction of DFAA in total amino acids in the seawater was relatively low; there were fewer reports on DI of DFAA in previous studies. In this study, we calculated the DI of DFAA in the water samples. The results showed that the degradation degree in the seawater of the euphotic layer and the mesopelagic layer of the research area varied significantly. The DI of many seawater samples in the two layers were negative with high absolute values, especially in the surface water above 100 m of station CTD01, indicating the organic matter in the seawater was very older. At the same depth, the DI in the seawater of stations CTD03 and CTD04 were positive in general. This obvious difference might attribute to the influence of the Yap Islands. Kobayashi (2000) reported the distance of the Yap Island Arc and the Yap Trench axis is abnormally short. Station CTD 01 is much closer to the Yap Islands than the other three stations, so it is more likely to be affected by the Yap Islands. As a small island, their effect on the adjacent sea area is limited. Therefore, the DI values in station CTD01 should reflect the input of old terrestrial organic matter around there. In the other three stations, fresh marine origin organic matter should constitute the major fraction of the organic matter. Below 1 000-m depth, most of the DI values were positive. These results suggested that the organic matter in the seawater of the bathypelagic layer, abyssal layer, and hadal zone was relatively fresh marine origin, and old terrestrial dissolved organic matter could not sink to the deeper layers, indicating the existence of seawater stratification in the study area prevented mixing processes between surface seawater and deep seawater below 1 000-m depth. For the dissolved organic matter in deep layers of the trench, two possible sources might be considered. One was from the releasing of sinking particulate organic matter to seawater, the other one was from transportation of dissolved organic matter in other regions by water current. For example, Liu et al. (2018) found that part of the water mass in the northern Yap Trench originated from the Lower Circumpolar Deep Water (LCPW). The low temperature of this water caused slow degradation of organic matter in it and then the relatively fresh dissolved organic matter was transported to the study area.
The DI values of the seawater from the five dive stations reflected the role of the funnel effect and bottom water current in the study area. Stations Dive 110, Dive 111, and Dive 112 were located in the east side of the study area, and close to or in the hadal zone, which was strongly affected by the funnel effect due to the V-shaped terrain of the trench (Yan et al., 2020). The funnel effect accelerated sedimentation and promoted enrichment of relatively fresh sinking organic matter in the sediment of the hadal zone. Then, resuspension of the sediment provided dissolved organic matter in the seawater of the sediment-seawater interface with positive DI values. Station Dive 109 is not in the V-shaped region. The sediment of this station tended to move downward and then decreased the sedimentation rate. The reserved sediment there became older. Thus, the dissolved organic matter from its resuspension had a higher degree of degradation, and the DI value became negative. Station Dive 113 was on the east side of the trench. Liu et al.'s (2018) results show that the water current in the abyssal layer and the hadal zone is strong enough to support sediment resuspension, and it is stronger on the west side than on the east side. They also confirmed that a part of the west propagating LCPW flowed through the East Mariana Basin and the East Caroline Basin to the study area. After a long-time and long-distance transportation, the old and high degraded organic matter carried by the LCPW was first deposited in the east side of the trench, causing the DI value of the sample from station Dive 113 turning negative.
On the other side, the molar percentages of amino acids are also related to their bioavailability. For example, Gly is abundant in the cell wall of diatoms, which is difficult to be degraded due to its inertness, resulting in selective preservation of Gly in the seawater during diagenesis process (Cowie and Hedges, 1992). Therefore, with the increase of the degradation degree, the molar percentage of Gly increase, and the DI become more negative (Dauwe et al., 1999; Dittmar et al., 2001). However, in this study, the molar percentage of Gly was positively correlated with DI (P < 0.001), which was negatively correlated with the degree of degradation of organic matter, indicating that the Gly in the DFAA of the northern region of the Yap Trench came from the new production of phytoplankton.
Additional to DI and molar percentages of amino acids, Tyr/Phe could also indicate early diagenesis of organic matter in a certain extent. In phytoplankton, Tyr/Phe is 1.2. The ratio decreases with the occurrence of degradation and diagenesis of dissolved organic matter (Emerson, 1985). In this study, more than 90% of the seawater samples had the ratio less than 1.2, suggesting that the organic matter in the study area was kept degrading from sea surface to the hadal zone. Statistical analysis showed that DI value was positively correlated with the values of Tyr/Phe. Both DI and Thr/Phe could effectively track the degradation of dissolved organic matter in the seawater of the north region of the Yap Trench.
4.2.2 Different degradation behavior of various amino acidsThe loading diagram of amino acids showed that the contribution of acidic amino acids (Asp, Glu) to the first principal component was negative, the contribution of neutral amino acids was positive, and the contribution of basic amino acids (His, Arg) was close to 0, indicating obvious clustering characteristics (Fig. 10). Previous studies on DI suggested that the first principal component obtained from PCA analysis of different organisms and sediments mainly represents the degradation of organic matter (Dauwe and Middelburg, 1998; Dauwe et al., 1999; Amon et al., 2001). The results of Fig. 10 showed that Ala had the largest contribution to the first principal component (PC1) of DFAA in the seawater, and other neutral amino acids (Gly, Ser, Thr, Tyr) also had a significant contribution to the PC1. The first and second principal components accounted for 22.8% and 21.6% of the variance, respectively. The positive and negative coefficients of single amino acids might lead to the change of DI value, suggesting that the concentrations of amino acids in different seawater samples are closely related to their sources and degradation processes (Table 8). For example, Gly had abundant sources and a significant degradation process in the study area, so its concentration varied significantly in different samples. Ala also had abundant sources, but its degradation was more significant than that of Gly. Then its concentrations in the seawater samples were significantly lower than that of Gly as a whole. Phe, Tyr, Leu, Ile, etc., as typical non-polar amino acids, were not abundant in the sources, but had high degree of degradation, so their concentrations were low in almost all the seawater samples. Yamashita and Tanoue (2003) found these amino acids were easily degradable in THAA. Our results further confirm they were also easily degradable components of DFAA.
![]() |
Fig.10 The diagram of amino acids load in seawater samples of the Yap Trench |
![]() |
In DFAA formation and consumption, the involvements of Chl a, DO, and DIN are necessary. Previous studies have shown that they might have some influence on the variations of concentrations of DFAA in the seawater (Jiang et al., 2007). Chl a is not only the major pigment for phytoplankton photosynthesis, but also an important indicator for phytoplankton biomass (Wang et al., 2015). Most of the metabolic activities of animals and algae in the ocean require the participation of DO. The growth of algae and the synthesis of proteins in marine organisms require involvement of DIN.
The concentrations of DFAA should be important to reflect the biomass of phytoplankton and metabolic processes in marine environment. However, from our results, there were no significant correlations between concentrations of DFAA and concentrations of Chl a, pH, and concentrations of DO and DIN. Generally, the pH value of seawater is significantly affected by CO2 in seawater. Concentration of Chl a and DO and pH values reflect the ability and strength of photosynthesis and respiration of seawater in some extent. Amino acids and protein are not direct products of photosynthesis, and are not direct energy sources of respiration. Therefore, it is reasonable not to find significant correlations between concentrations of DFAA and the parameters Chl a, DO, and pH. Poulet et al. (1991) found that in shelf seawater, if the concentration of DFAA was below 0.65 μmol/L, the correlation between it and the concentration of Chl a was not significant. In our study, the average concentration of DFAAs was 0.47±0.36 μmol/L, and most of the values were below 0.65 μmol/L, so our results agreed with theirs. However, the effects of different environmental factors on DFAA are complex in the marine environment. The feature of DFAA in various water layers of the Yap Trench is the result of multiple environmental factors, and any single factor have no prominent effect on the concentration and composition of DFAA (Qiao et al., 2016).
4.4 Similarities and differences in biochemical behavior of various amino acids of DFAAA correlation matrix analysis was performed on the relative content of DFAA in the seawater samples (Table 9). The data indicated that there were significant positive correlations between 9 pairs of amino acids in the DFAA (Glu-Asp, Arg-His, AlaSer, Ala-Thr, Tyr-Arg, Met-His, Phe-His, Phe-Arg, and Phe-Tyr). This kind of correlation suggested that they likely shared similar activity in seawater and might be affected by similar biogeochemical processes. For example, Asp and Glu belong to acidic amino acids, Arg and His belong to basic amino acids, and Phe and Tyr belong to aromatic amino acids. The only difference between Ala and Ser is that H in methyl group is replaced by -OH group. The molecular weight of Tyr and Arg, Met and His, Phe and His, and Phe and Arg are similar. In fact, the average molecular weight of the twenty amino acids ubiquitous in organisms is about 128. The molecular weights of Tyr, Arg, Met, His, and Phe are all higher than this value. Their positive correlation with each other suggested that the role of molecular size on their biogeochemical processes should not be ignored. Ten pairs of amino acids were negatively correlated with each other (Ser-Glu, Ser-Asp, His-Ser, Gly-Asp, Gly-Glu, Gly-His, Ala-Asp, Ala-Glu, Val-Asp, and Leu-Met). Since Glu is prone to transamination, it is reasonable to find negative correlations between Glu and Ser, Gly, and Ala. As typical non-polar amino acids, their negative correlation Ala and Val with acidic amino acids Asp and Glu reflected the difference between their fundamental chemical properties. The molecular weights of Ser, Gly, Ala and Val are less than 128, and Asp, His, and Glu are higher than 128. The negative correlations between the 9 pairs of amino acids (except Leu-Met) might indicate the differences of their molecular size, further supporting the opinion that the molecular size could affect the biogeochemical processes of various acids. However, the factors affecting the concentrations of different amino acids in seawater were complex, including protein synthesis and hydrolysis, synthesis and degradation of amino acids, photosynthesis, respiration, water temperature, microbial utilization, etc. The significance of the correlation between concentrations of different amino acids needs to be further explored.
![]() |
For the first time, we conducted a direct quantitative study on the DFAA in the seawater of the northern region of the Yap Trench from sea surface to the hadal zone, providing evidence for understanding ocean nitrogen and carbon cycles in the abyssal and hadal seawater. Overall, the vertical variation profiles of DFAA concentrations in the seawater tended to decrease with the depth at the four CTD stations, but the variations of DFAA concentrations were complex above the mesopelagic layer of the trench (< 1 000 m). Above 1 000-m depth, the sources of dissolved organic matter had both terrestrial and marine origins. Below 1 000-m depth, the sources were dominated by marine origin. The concentrations of DFAA in the study area were affected by photosynthesis and respiration, and terrigenous input had a significant influence above the euphotic layer and mesopelagic layer. The enrichment of DFAA in the mesopelagic layer of the trench was related to dramatic changes of the environmental factors from the euphotic layer to the mesopelagic layer, such as the abundance of the microorganism, the oxygen minimum layer and temperature, and the organic matter degradation. The variation of DFAA concentration in the bathypelagic and abyssal layer was the combined results of organic matter transport, physical mixing of water mass, ocean circulation and "funnel effect". At the seawater-sediment interface, the concentration of DFAA on the western side of the trench was higher than that on the eastern side, attributing to the roles of sediment resuspension and LCPW. In general, the dominant amino acids of DFAA in different water layers of the northern region of the Yap Trench were similar, and concentrations of some amino acids have a significant correlation, indicating the continuity of similar biogeochemical processes from sea surface to the hadal zone. The characteristics of DFAA in the study area are the results of the effect of different environmental factors, and any single factor had no prominent effect on the feature of DFAA in the seawater of the trench. The cyclic transformation of DFAA throughout the Yap Trench will be helpful to further understand the global cycle of organic nitrogen and carbon in the oceans.
6 DATA AVAILABILITY STATEMENTThe datasets generated during the current study are available from the corresponding author on reasonable request.
Alyuruk H, Kontas A. 2021. Dissolved free, total and particulate enantiomeric amino acid levels in eutrophic and oligotrophic parts of a semi-enclosed bay (İzmir, Aegean Sea). Regional Studies in Marine Science, 44: 101750.
DOI:10.1016/j.rsma.2021.101750 |
Amon R M W, Fitznar H P, Benner R. 2001. Linkages among the bioreactivity, chemical composition, and diagenetic state of marine dissolved organic matter. Limnology and Oceanography, 46(2): 287-297.
DOI:10.4319/lo.2001.46.2.0287 |
Behrends B, Liebezeit G. 1999. Particulate amino acids in Wadden Sea waters-seasonal and tidal variations. Journal of Sea Research, 41(1-2): 141-148.
DOI:10.1016/S1385-1101(98)00044-6 |
Braven J, Butler E I, Chapman J, et al. 1995. Changes in dissolved free amino acid composition in sea water associated with phytoplankton populations. Science of the Total Environment, 172(2-3): 145-150.
DOI:10.1016/0048-9697(95)04784-0 |
Burdige D J. 1991. Microbial processes affecting alanine and glutamic acid in anoxic marine sediments. FEMS Microbiology Letters, 85(3): 211-231.
DOI:10.1111/j.1574-6968.1991.tb04728.x |
Carney R S. 2005. Zonation of deep biota on continental margins. Oceanography and Marine Biology: An Annual Review, 43: 211-278.
|
Chen Y, Yang G P. 2010. Study on dissolved free amino acid(DFAA) in the surface water of the North Yellow Sea. Periodical of Ocean University of China, 40(7): 93-98.
(in Chinese with English abstract) DOI:10.3969/j.issn.1672-5174.2010.07.016 |
Clark M E, Jackson G A, North W J. 1972. Dissolved free amino acids in southern California coastal water. Limnology and Oceanography, 17(5): 749-758.
DOI:10.4319/lo.1972.17.5.0749 |
Cowie G L, Hedges J I. 1992. Sources and reactivities of amino acids in a coastal marine environment. Limnology and Oceanography, 37(4): 703-724.
DOI:10.4319/lo.1992.37.4.0703 |
Cowie G L, Hedges J I. 1994. Biochemical indicators of diagenetic alteration in natural organic matter mixtures. Nature, 369(6478): 304-307.
DOI:10.1038/369304a0 |
Dauwe B, Middelburg J J, Herman P M J, et al. 1999. Linking diagenetic alteration of amino acids and bulk organic matter reactivity. Limnology and Oceanography, 44(7): 1809-1814.
DOI:10.4319/lo.1999.44.7.1809 |
Dauwe B, Middelburg J J. 1998. Amino acids and hexosamines as indicators of organic matter degradation state in North Sea sediments. Limnology and Oceanography, 43(5): 782-798.
DOI:10.4319/lo.1998.43.5.0782 |
Davis J, Kaiser K, Benner R. 2009. Amino acid and amino sugar yields and compositions as indicators of dissolved organic matter diagenesis. Organic Geochemistry, 40(3): 343-352.
DOI:10.1016/j.orggeochem.2008.12.003 |
Dickson A G, Sabine C L, Christian J R. 2007. Guide to Best Practices for Ocean CO2 Measurements. North Pacific Marine Science Organization, Sidney, Canada.
|
Dittmar T, Fitznar H P, Kattne G. 2001. Origin and biogeochemical cycling of organic nitrogen in the eastern Arctic Ocean as evident from D- and L-amino acids. Geochimica et Cosmochimica Acta, 65(22): 4103-4114.
DOI:10.1016/S0016-7037(01)00688-3 |
Emerson S. 1985. Organic carbon preservation in marine sediments. In: Sundquist E T, BroeckerW S eds. The Carbon Cycle and Atmospheric CO2: Natural Variations Archean to Present. American Geophysical Union, Washington. p. 78-87.
|
Fujiwara T, Tamura C, Nishizawa A, et al. 2000. Morphology and tectonics of the Yap Trench. Marine Geophysical Researches, 21(1-2): 69-86.
DOI:10.1023/A:1004781927661 |
Guo C Y, Yang Z, Chen J F, et al. 2018. A preliminary study on the food resources and trophic levels of the benthic community in the Yap Trench based on stable carbon and nitrogen isotopes. Haiyang Xuecbao, 40(10): 51-60.
(in Chinese with English abstract) DOI:10.3969/j.issn.0253-4193.2018.10.006 |
Hamilton E I. 1984. A manual of chemical & biological methods for seawater analysis: T. R. Parsons, Y. Maita & C. M. Lalli. Pergamon Press, Oxford, 1984. 184pp, 7 illustrations and 120 literature references. Hard-backed £12.25. ISBN 0 08 030288 2. Soft-backed £5.50. ISBN 0 08 030287 4. Marine Pollution Bulletin, 15(11): 419-420, https://doi.org/10.1016/0025-326X(84)90262-5.
|
Hawkins J, Batiza R. 1977. Metamorphic rocks of the Yap arctrench system. Earth and Planetary Science Letters, 37(2): 216-229.
DOI:10.1016/0012-821X(77)90166-2 |
Hayashi T, Suitani Y, Murakami M, et al. 1986. Protein and amino acid compositions of five species of marine phytoplankton. Nippon Suisan Gakkaishi, 52(2): 337-343.
DOI:10.2331/suisan.52.337 |
Huang Y H, Sun C J, Yang G P, et al. 2020. Geochemical characteristics of hadal sediment in the northern Yap Trench. Journal of Oceanology and Limnology, 38(3): 650-664.
DOI:10.1007/s00343-019-9010-3 |
Hubberten U, Lara R J, Kattner G. 1995. Refractory organic compounds in polar waters: relationship between humic substances and amino acids in the Arctic and Antarctic. Journal of Marine Research, 53(1): 137-149.
DOI:10.1357/0022240953213322 |
Jiang S C, Zheng A R, Quan Y Q. 2007. Distribution of dissolved free amino acids and its relationships with environment factors in winter in Beibu Gulf. Journal of Xiamen University (Natural Science), 46(S1): 43-48.
(in Chinese with English abstract) DOI:10.3321/j.issn:0438-0479.2007.z1.010 |
Jørgensen N O G, Kroer N, Coffin R B, et al. 1993. Dissolved free amino acids, combined amino acids, and DNA as sources of carbon and nitrogen to marine bacteria. Marine Ecology Progress Series, 98(1-2): 135-148.
DOI:10.3354/meps098135 |
Kaneko I, Takatsuki Y, Kamiya H, et al. 1998. Water property and current distributions along the WHP-P9 section(137°-142°) in the western North Pacific. Journal of Geophysical Research: Oceans, 103(C6): 12959-12984.
DOI:10.1029/97JC03761 |
Keeling C D, Revelle R. 1985. Effects of El Nino/southern oscillation on the atmospheric content of carbon dioxide. Meteoritics, 20: 437-450.
|
Kirchman D, Hodson R. 1984. Inhibition by peptides of amino acid uptake by bacterial populations in natural waters: Implications for the regulation of amino acid transport and incorporation. Applied and Environmental Microbiology, 47(4): 624-631.
DOI:10.1128/aem.47.4.624-631.1984 |
Klevenz V, Sumoondur A, Ostertag-Henning C, et al. 2010. Concentrations and distributions of dissolved amino acids in fluids from Mid-Atlantic Ridge hydrothermal vents. Geochemical Journal, 44(5): 387-397.
DOI:10.2343/geochemj.1.0081 |
Kobayashi K. 2000. Horizontally-moving subducted slab may generate enigmatic features of the Palau and Yap TrenchArcs. Proceedings of the Japan Academy, Series B, 76(9): 133-138.
DOI:10.2183/pjab.76.133 |
Kuznetsova M, Lee C, Aller J, et al. 2004. Enrichment of amino acids in the sea surface microlayer at coastal and open ocean sites in the North Atlantic Ocean. Limnology and Oceanography, 49(5): 1605-1619.
DOI:10.4319/lo.2004.49.5.1605 |
Lee C, Bada J L. 1975. Amino acids in equatorial Pacific Ocean water. Earth and Planetary Science Letters, 26(1): 61-68.
DOI:10.1016/0012-821X(75)90177-6 |
Lee C, Cronin C. 1982. The vertical flux of particulate organic nitrogen in the sea: decomposition of amino acids in the Peru upwelling area and the equatorial Atlantic. Journal of Marine Research, 40(1): 227-251.
|
Li J. 2007. Analysis and distribution of dissolved free amino acids in the East China Sea and Yellow Sea. Ocean University of China, Qingdao, China. p. 1-64. (in Chinese with English abstract)
|
Lindroth P, Mopper K. 1979. High Performance Liquid chromatographic determination of subpicomole amounts of amino acids by precolumn fluorescence derivatization with o-phthaldialdehyde. Analytical Chemistry, 51(11): 1667-1674.
DOI:10.1021/ac50047a019 |
Liu S T, Liu Z F. 2018. Free extracellular enzymes dominate initial peptide hydrolysis in coastal seawater. Marine Chemistry, 199: 37-43.
DOI:10.1016/j.marchem.2018.01.005 |
Liu Y Z, Liu X H, Lv X Q, et al. 2018. Watermass properties and deep currents in the northern Yap Trench observed by the Submersible Jiaolong system. Deep Sea Research Part I: Oceanographic Research Papers, 139: 27-42.
DOI:10.1016/j.dsr.2018.06.001 |
Luo M, Gieskes J, Chen L Y, et al. 2019. Sources, Degradation, and transport of organic matter in the New Britain shelf-trench continuum, Papua New Guinea. Journal of Geophysical Research: Biogeosciences, 124(6): 1680-1695.
DOI:10.1029/2018JG004691 |
Luo M, Glud R N, Pan B B, et al. 2018. Benthic carbon mineralization in hadal trenches: Insights from in situ determination of benthic oxygen consumption. Geophysical Research Letters, 45(6): 2752-2760.
DOI:10.1002/2017GL076232 |
Matsumoto K, Furuya K, Kawano T. 2004. Association of picophytoplankton distribution with ENSO events in the equatorial Pacific between 145°E and 160°W. Deep Sea Research Part I: Oceanographic Research Papers, 51(12): 1851-1871.
DOI:10.1016/j.dsr.2004.07.015 |
Matsumoto K, Uematsu M. 2005. Free amino acids in marine aerosols over the western North Pacific Ocean. Atmospheric Environment, 39(11): 2163-2170.
DOI:10.1016/j.atmosenv.2004.12.022 |
Middelboe M, Borch N H, Kirchman D L. 1995. Bacterial utilization of dissolved free amino acids, dissolved combined amino acids and ammonium in the Delaware Bay Estuary: effects of carbon and nitrogen limitation. Marine Ecology Progress Series, 128(1): 109-120.
DOI:10.3354/meps128109 |
Mopper K, Lindroth P. 1982. Diel and depth variations in dissolved free amino acids and ammonium in the Baltic Sea determined by shipboard HPLC analysis. Limnology and Oceanography, 27(2): 336-347.
DOI:10.4319/lo.1982.27.2.0336 |
Mopper K, Zika R G. 1987. Free amino acids in marine rains: evidence for oxidation and potential role in nitrogen cycling. Nature, 325(6101): 246-249.
DOI:10.1038/325246a0 |
Pettine M, Patrolecco L, Manganelli M, et al. 1999. Seasonal variations of dissolved organic matter in the northern Adriatic Sea. Marine Chemistry, 64(3): 153-169.
DOI:10.1016/S0304-4203(98)00071-1 |
Poulet S A, Williams R, Conway D V P, et al. 1991. Cooccurrence of copepods and dissolved free amino acids in shelf sea waters. Marine Biology, 108(3): 373-385.
DOI:10.1007/BF01313646 |
Preston T, Bury S, Mcmeekin B, et al. 1996. Isotope Dilution analysis of combined nitrogen in natural waters: Ⅱ. Amino Acids. Rapid Communications in Mass Spectrometry, 10(8): 965-968.
DOI:10.1002/(SICI)1097-0231(19960610)10:8<965::AID-RCM536>3.0.CO;2-O |
Qiao L, Mi T Z, Zhen Y, et al. 2016. Distribution characteristics and seasonal changes of dissolved amino acids in the brown tide outbreak area in Qinhuangdao sea. Periodical of Ocean University of China, 46(5): 95-103.
(in Chinese with English abstract) DOI:10.16441/j.cnki.hdxb.20150259 |
Romankevich A E. 1984. Geochemistry of Organic Matter in the Ocean. Springer-Verlag, Berlin, Germany, https://doi.org/10.1007/978-3-642-49964-7.
|
Sabadel A J M, Browning T J, Kruimer D, et al. 2017. Determination of picomolar dissolved free amino acids along a South Atlantic transect using reversed-phase high-performance liquid chromatography. Marine Chemistry, 196: 173-180.
DOI:10.1016/j.marchem.2017.09.008 |
Scheller E. 2001. Amino acids in dew-origin and seasonal variation. Atmospheric Environment, 35(12): 2179-2192.
DOI:10.1016/s1352-2310(00)00477-5 |
Shi D, Yang G P, Sun Y, et al. 2017. Spatiotemporal variation of dissolved carbohydrates and amino acids in Jiaozhou Bay, China. Chinese Journal of Oceanology and Limnology, 35(2): 383-399.
DOI:10.1007/s00343-016-5218-7 |
Sohrin R, Imazawa M, Fukuda H, et al. 2010. Full-depth profiles of prokaryotes, heterotrophic nanoflagellates, and ciliates along a transect from the equatorial to the subarctic central Pacific Ocean. Deep Sea Research Part II: Topical Studies in Oceanography, 57(16): 1537-1550.
DOI:10.1016/j.dsr2.2010.02.020 |
Somville M, Billen G. 1983. A method for determining exoproteolytic activity in natural waters. Limnology and Oceanography, 28(1): 190-193.
DOI:10.4319/lo.1983.28.1.0190 |
Song Y D, Ma X C, Zhang G X, et al. 2016. Heat flow insitu measurement at Yap Trench of the Western Pacific. Marine Geology & Quaternary Geology, 36(4): 51-56.
(in Chinese with English abstract) DOI:10.16562/j.cnki.0256-1492.2016.04.006 |
Strickland J D H, Parsons T R. 1972. A Practical Handbook of Seawater Analysis. Fisheries Research Board of Canada Bulletin. 2nd edn. Fisheries Board of Canada, Ottawa. p. 310.
|
Sun Y. 2012. Distribution and composition of dissolved amino acids in the South Yellow Sea and Jiaozhou Bay. Ocean University of China, Qingdao, China. p. 1-59. (in Chinese with English abstract)
|
Svensson E, Skoog A, Amend J P. 2004. Concentration and distribution of dissolved amino acids in a shallow hydrothermal system, Vulcano Island (Italy). Organic Geochemistry, 35(9): 1001-1014.
DOI:10.1016/j.orggeochem.2004.05.005 |
Veuger B, Middelburg J J, Boschker H T S, et al. 2004. Microbial uptake of dissolved organic and inorganic nitrogen in Randers Fjord. Estuarine, Coastal and Shelf Science, 61(3): 507-515.
DOI:10.1016/j.ecss.2004.06.014 |
Wang P, Chen Y, Yang G P, et al. 2015. Distribution and composition of dissolved amino acids in the East China Sea and the Yellow Sea during spring. Marine Environmental Science, 34(2): 217-224.
(in Chinese with English abstract) DOI:10.13634/j.cnki.mes.2015.02.011 |
Wedyan M A, Preston M R. 2008. The coupling of surface seawater organic nitrogen and the marine aerosol as inferred from enantiomer-specific amino acid analysis. Atmospheric Environment, 42(37): 8698-8705.
DOI:10.1016/j.atmosenv.2008.04.038 |
Wishner K F, Ashjian C J, Gelfman C, et al. 1995. Pelagic and benthic ecology of the lower interface of the Eastern Tropical Pacific oxygen minimum zone. Deep Sea Research Part I: Oceanographic Research Papers, 42(1): 93-115.
DOI:10.1016/0967-0637(94)00021-J |
Wu B, Li D, Zhao J, et al. 2018. Vertical distribution of sedimentary organic carbon in the Yap Trench and its implications. China Environmental Science, 38(9): 3502-3511.
(in Chinese with English abstract) DOI:10.19674/j.cnki.issn1000-6923.2018.0379 |
Xiao W J, Xu Y P, Haghipour N, et al. 2020. Efficient sequestration of terrigenous organic carbon in the New Britain Trench. Chemical Geology, 533: 119446.
DOI:10.1016/j.chemgeo.2019.119446 |
Xu N, Liu J Y, Lai H Y, et al. 2013. Uptake and utilization characteristics of Prorocentrum donghaiense, Phaeoecystis globosa, Karenia mikimotoi and Skeletonema costatum for dissolved free amino acids. Acta Scientiae Circumstantiae, 33(4): 1058-1065.
(in Chinese with English abstract) DOI:10.13671/j.hjkxxb.2013.04.007 |
Yamashita Y, Tanoue E. 2003. Distribution and alteration of amino acids in bulk DOM along a transect from bay to oceanic waters. Marine Chemistry, 82(3-4): 145-169.
DOI:10.1016/S0304-4203(03)00049-5 |
Yan Y X, Sun C J, Huang Y H, et al. 2020. Distribution characteristics of lipids in hadal sediment in the Yap Trench. Journal of Oceanology and Limnology, 38(3): 634-649.
DOI:10.1007/s00343-019-8120-2 |
Yue X A, Yan Y X, Ding H B, et al. 2018. Biological geochemical characteristics of the sediments in the Yap trench and its oceanographic significance. Periodical of Ocean University of China, 48(3): 88-96.
(in Chinese with English abstract) DOI:10.16441/j.cnki.hdxb.20170145 |
Zhang P Y, Chen Y, Yang G P. 2015. Composition and distribution of dissolved and particulate amino acids in seawater of East China Sea in autumn. Oceanologia et Limnologia Sinica, 46(2): 329-339.
(in Chinese with English abstract) DOI:10.11693/hyhz20140500151 |
Zhang P Y, Yang G P, Chen Y, et al. 2016. Temporal and spatial variations of particulate and dissolved amino acids in the East China Sea. Marine Chemistry, 186: 133-144.
DOI:10.1016/j.marchem.2016.09.004 |